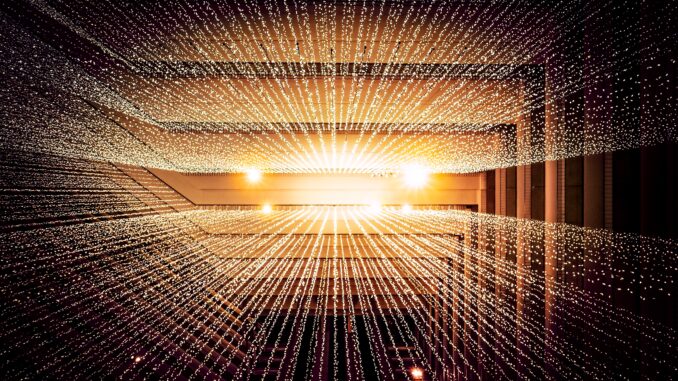
By Vipin Jain
(What follows is Part 1 of Quantum Computing Demystified. Part 2 will appear tomorrow.)
Executive Summary
Quantum computing represents a profound shift in computational technology, heralding a new era of problem-solving across scientific research, and industry applications, and addressing global challenges. This white paper explores the transition from classical computing paradigms to quantum computing, emphasizing its potential to revolutionize various sectors.
By delving into the theoretical foundations and practical applications of quantum technologies, I aim to illuminate their transformative impact and the strategic importance for businesses and IT leaders.
As quantum computing moves from experimental labs to real-world applications, it promises significant advancements in drug discovery, materials science, and cybersecurity, among others. Its unique ability to process complex data sets and enhance information security makes it a critical asset for future business strategies and maintaining a competitive edge.
Understanding quantum computing is crucial for business and IT leaders as they navigate the integration of quantum technologies into industry practices. This white paper serves as a guide, providing insights into the strategic significance of quantum computing and how it can shape the future of business and technology. We aim to equip executives with the knowledge to lead their organizations into the quantum era, driving innovation and securing a competitive advantage in the rapidly evolving digital landscape.
Introduction to Quantum Computing
Quantum computing heralds a transformative era in information processing, poised to redefine our approach to solving complex problems that remain intractable for classical computing systems. This introduction demystifies the fundamental principles of quantum computing, contrasts it with classical computing paradigms, and explores its burgeoning technological landscape and potential to reshape various fields.
Contrasting Quantum and Classical Computing
Classical computing, from its inception with mechanical calculators to the advanced supercomputers of today, operates on binary bits. These bits, constrained to either 0 or 1, form the backbone of classical computation but encounter limitations with multidimensional and complex problem-solving.
Quantum computing introduces a paradigm shift through the use of quantum bits, or qubits. Unlike their classical counterparts, qubits thrive on the principle of superposition, allowing them to embody multiple states simultaneously. This capability enables quantum computers to parallel process a multitude of possibilities, significantly accelerating complex computations.
Entanglement, a distinctive quantum phenomenon, further elevates quantum computing. Entangled qubits exhibit a profound correlation, where the state of one instantly influences another, regardless of distance, fostering a new level of efficiency and coordination in computational tasks.
While quantum computing offers exponential speedups for certain types of problems, it’s not universally superior to classical computing for all tasks. The challenge lies in identifying specific applications where quantum computing can provide a distinct advantage, such as complex simulations, cryptography, and optimization problems while recognizing areas where classical computing remains the most efficient and practical solution.
Core Components of Quantum Computing
Quantum computing’s essence lies in the qubit’s power to transcend classical bit limitations through superposition and entanglement. These properties facilitate a quantum leap in processing capabilities, allowing for the exploration of computational realms previously deemed unattainable.
Qubits are the core units of quantum information in quantum computing, capable of existing in multiple states simultaneously thanks to quantum superposition. Unlike classical bits that represent either 0 or 1, qubits leverage the principles of quantum mechanics to perform complex calculations more efficiently, encoded in physical properties like photon polarization or electron spin. This allows quantum computers to achieve unprecedented computational capabilities.
Superposition: This principle grants a qubit the ability to be in a blend of all its possible states at once, permitting simultaneous execution of numerous calculations.
Entanglement: A quantum interconnection between qubits where the condition of one qubit instantaneously aligns with another, enabling complex computations unreachable by classical computers.
Additional Terms Used in the Scope of Quantum Computing
For tech-savvy executives exploring the potential of quantum computing within their industries, understanding the following refined set of terms will be invaluable:
Fault-tolerant Quantum Computing: These are advanced quantum computers capable of operating reliably across a wide range of applications. They are essential for achieving quantum advantage through complex algorithms like Shor’s algorithm. However, the widespread deployment of fault-tolerant systems is a future goal, challenged by the need for intricate error correction to manage qubit fragility.
Quantum Noise and Stability: Today’s quantum computers are akin to the early days of radio, with “quantum noise” playing a role similar to static in AM/FM radios. This noise significantly impacts the stability and reliability of qubits, posing a greater challenge than noise in traditional computing systems.
Quantum Advantage and Speedup: Quantum advantage occurs when quantum computers solve problems beyond the reach of classical systems within a practical timeframe, often leveraging exponential speedup in performance. Quantum speedup, the benchmark for this advantage, highlights the efficiency gains quantum computing offers, especially as the number of qubits increases.
Quantum Economic Impact: Beyond theoretical and technical achievements, quantum computing achieves economic advantage when it solves problems more efficiently or uniquely compared to classical computing, potentially transforming industries with quadratic or polynomial improvements in processing times.
Quantum Entanglement and Cryptography: Understanding quantum entanglement is key to grasping how quantum computers achieve extraordinary computational power by interlinking qubits in a state where the action on one instantly affects another, regardless of distance. This principle is foundational to quantum cryptography, which promises to revolutionize data security by making communication virtually unbreakable, a critical aspect as quantum computing evolves.
Current State of Quantum Technology
The quantum computing landscape is marked by the development and application of two main types of quantum computers Quantum annealers and universal gate quantum computers.
Quantum Annealers are specialized devices aimed at solving optimization problems by identifying the lowest energy state of a system. This capability finds significant applications in sectors like finance, logistics, and materials science, where optimization is key.
Universal Gate quantum computers offer a broader range of computational abilities. They operate through programmable gates that control qubits, making them versatile tools for various computational tasks beyond optimization.
Despite the significant promise quantum computing holds, the technology is still in the early stages of development. The field faces challenges such as enhancing qubit stability, reducing error rates, and improving scalability. Nonetheless, progress in quantum error correction and algorithm development is propelling quantum computing towards achieving practical, fault-tolerant systems.
Quantum computers possess the potential to execute algorithms more efficiently than classical computers, potentially offering a “shorter path” to solving problems. However, their typically slower processing speeds mean that, in some cases, it could take longer to reach a solution. This paradox underscores the importance of a strategic approach in determining when the use of quantum computing is most advantageous.
The pursuit of quantum advantage, defined as the ability of quantum computers to solve problems unattainable by classical computers, remains a long-term goal, with some projections estimating its achievement by 2030. However, the concept of quantum advantage may overshadow the practical benefits that quantum computers can offer as they become cost-competitive with classical computing resources.
Quantum economic advantage, a new benchmark termed is proposed. This benchmark is met when a quantum computer can solve a problem more quickly than a classical computer of comparable price, under two conditions:
- Feasibility (the existence of a quantum computer powerful enough to tackle the problem)
- Algorithmic advantage (the superior speed of a quantum computer in performing a specific task)
The intersection of feasibility and algorithmic advantage defines the quantum economic advantage, emphasizing the need for strategic investment in quantum computing based on the nature and duration of the computational challenges faced.
Advancements in Quantum Technology
The quantum computing arena is characterized by swift progress and varied methodologies, such as quantum annealers tailored for optimization challenges and universal gate quantum computers, which hold promise for a broader application spectrum. Nonetheless, the journey ahead is filled with hurdles, including enhancing qubit stability, minimizing error rates, and devising algorithms that fully exploit quantum mechanics for real-world problem-solving. This evolving landscape underscores a pivotal shift in computational theory and practice, signaling a future where quantum computing’s full potential can be unleashed.
Quantum Computing readiness for prime time
Quantum computing is at an early but rapidly advancing stage, marked by significant milestones and ambitious goals. IBM’s introduction of the Osprey, a 433-qubit quantum computer, exemplifies this progress, with aims to escalate to a 100,000-qubit system within a decade. Google’s ambitious target reaches even further, aiming for a million qubits by the decade’s end. The quantum computing arena is also populated by other key players like D-Wave Systems, IonQ, Rigetti Computing, Honeywell, Microsoft, Intel, and PsiQuantum, many of which are extending their services through cloud platforms. The market’s growth trajectory is notable, with forecasts predicting an increase from $928.8 million to $6.5 billion by 2030, reflecting a 32.1% compound annual growth rate.
Quantum computers offer unparalleled advantages in solving complex problems across various domains, from material simulation and drug discovery to optimization in manufacturing and finance. However, the practical deployment of quantum computing on a broad scale face hurdles. Estimates suggest that while 5,000 quantum computers may be operational by 2030, the comprehensive hardware and software needed for tackling the most intricate problems might not be fully developed until 2035 or later. This underscores the necessity for organizations to begin strategizing on potential applications of quantum technology.
A collaboration between MIT researchers and Accenture has led to the creation of a framework designed to assist executives in assessing quantum computing’s applicability to their problem-solving needs. The framework emphasizes the importance of discerning the circumstances under which quantum computing offers a competitive edge over classical computing, considering that quantum’s superiority is highly problem specific.
While quantum computing has achieved notable benchmarks like Google’s claim to quantum supremacy in 2019, it’s important to recognize its limitations. Quantum computers excel at tasks involving quantum simulation, certain optimizations, and problems where quantum algorithms significantly outpace classical approaches. Conversely, classical computers currently surpass quantum computers in general computing tasks, algorithmic efficiency, and reliability, owing to their advanced development, lower error rates, and optimized algorithms.
In essence, quantum computing holds transformative potential for specific problem domains, particularly those involving complex simulations and optimizations where quantum algorithms can dramatically accelerate processes. Nonetheless, for everyday computing needs and most business applications, classical computing remains the superior choice due to its proven reliability, efficiency, and the optimization of existing algorithms. The field of quantum computing is burgeoning, with distinct niches where it excels, yet it is not a one-size-fits-all solution for all computing requirements.
Author
Vipin Jain is the founder and Chief Architect at Transformation Enablers Inc. (TXE.AI) in Chantilly, Virginia, USA. With over three decades of technology management and solution delivery experience, Jain specializes in developing and implementing IT strategies and plans aligned with business goals, solution architecture, digital transformation roadmaps, portfolio optimization, and the deployment of global business solutions.
Before founding TXE, Jain held various executive roles at Microsoft, Accenture, HPES, and TEKsystems, advising Fortune 500 companies and US federal agencies on innovative solutions. His career also includes executive positions at AIG, Merrill Lynch, Citibank, First Chicago, and Empire Blue Cross Blue Shield, where he managed technology for enterprise-wide initiatives and conducted due diligence for potential acquisitions.
Jain’s experience spans large and small commercial firms, US federal and state government agencies, and management consulting, covering multiple business sectors like banking, brokerage, insurance, healthcare, pharmaceuticals, energy, and government. He is actively involved in industry groups such as ACT-IAC and SIMCAC, focusing on public sector digital and IT transformation, big data, and cloud technologies, in addition to being a member of IASA Global and the Chief Architect Forum.
Vipin can be reached at Vipin@txe.ai.